1.5 Metabolism
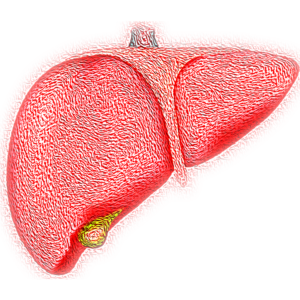
Once a drug has been absorbed and distributed in the body, it will then be broken down by a process known as . The breakdown of a drug molecule usually involves two steps that take place primarily in the body’s chemical processing plant: the liver. (See Figure 1.5[1] for an image of a human liver.) Everything that enters the bloodstream—whether swallowed, injected, inhaled, absorbed through the skin, or produced by the body itself—is carried to this largest internal organ.
The biotransformations that take place in the liver are performed by the liver enzymes. Every one of your cells has a variety of enzymes, and each enzyme specializes in a particular job. Some enzymes break molecules apart, while others link small molecules into long chains. With drugs, the first step in metabolizing occurs through a process known as the first pass effectno post, in which orally administered drugs are broken down in the liver and intestines. This makes the substance easier to excrete in the urine. Medications made of protein that are swallowed or otherwise absorbed in the GI tract may quickly be deactivated by enzymes as they pass through the stomach and duodenum. If the drug enters the blood from the intestines, part of it will be broken down by liver enzymes, known as the first pass effect, and some of it will escape to the general circulation to either be protein-bound (inactive) or stay free (and create an action at a receptor site). Thus, several doses of an oral medication may be needed to maintain enough active free drug in the circulation to exert the desired effect.
Many of the products of enzymatic breakdown, which are called metabolites, are less chemically active than the original molecule. For this reason, scientists refer to the liver as a “detoxifying” organ. However, rather than being destroyed by liver enzymes, a few drugs are metabolized into an active form of an intended drug called a “prodrug.” Prodrugs have chemical activities of their own—sometimes as powerful as those of the original drug. When prescribing certain drugs, healthcare providers must take into account these added effects. Once liver enzymes are finished working on a medicine, the now-inactive drug undergoes the final stage of its time in the body – excretion – as it exits via the urine or feces. [2]
Lifespan Considerations
Neonate & Pediatric: The developing liver in infants and young children produces decreased levels of microsomal enzymes. This may result in a decreased ability of the young child or neonate to metabolize medications. In contrast, older children may experience increased metabolism and require higher doses of medications once the hepatic enzymes are fully produced.[3]
Older Adult: Hepatic metabolism may experience a significant decline in the older adult. As a result, dosages should be adjusted according to the patient’s liver function and anticipated metabolic rate. First-pass metabolism is also decreased with aging; therefore, older adults may have higher “free” criculating drug concentrations and be at higher risk for side effects and toxicities.[4]
Critical Thinking Activity 1.5a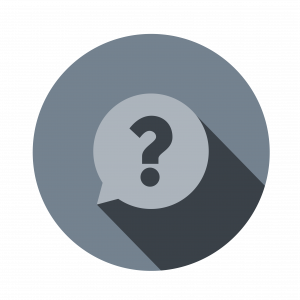
Metabolism can be influenced by many factors within the body. If a patient has liver damage, the patient may not be able to breakdown (metabolize) medications as efficiently. Dosages are calculated according to the liver’s ability to metabolize and the kidney’s ability to excrete.
When caring for a patient with cirrhosis, how does this condition impact the dosages prescribed for the patient?
Note: Answers to the Critical Thinking activities can be found in the “Answer Key” sections at the end of the book.
Did you know the power of grapefruit juice?
A Juicy Story[5]
Did you know that, in some people, a single glass of grapefruit juice can alter levels of drugs used to treat allergies, heart diseases, and infections? Fifteen years ago, pharmacologists discovered this “grapefruit juice effect” by luck, after giving volunteers grapefruit juice to mask the taste of a medicine. Nearly a decade later, researchers figured out that grapefruit juice affects the metabolizing rates of some medicines by lowering levels of a drug-metabolizing enzyme, called CYP3A4 (part of the CYP450 family of drug-binding enzymes), in the intestines.
More recently, Paul B. Watkins of the University of North Carolina at Chapel Hill discovered that other juices like Seville (sour) orange juice—but not regular orange juice—have the same effect on the liver’s ability to metabolize using enzymes. Each of ten people who volunteered for Watkins’ juice-medicine study took a standard dose of felodopine (Plendil), a drug used to treat high blood pressure, diluted in grapefruit juice, sour orange juice, or plain orange juice. The researchers measured blood levels of Plendil at various times afterward. The team observed that both grapefruit juice and sour orange juice increased blood levels of Plendil, as if the people had received a higher dose. Regular orange juice had no effect. Watkins and his coworkers have found that a chemical common to grapefruit and sour oranges, dihydroxybergamottin, is likely the molecular culprit. Thus, when taking medications that use the CYP3A4 enzyme to metabolize, patients are advised to avoid grapefruit juice and sour orange juice. [6]
- 'Liver Hepatic Organ Jaundice Bile Fatty Liver - Liver' by VSRao is licensed under CC0 ↵
- This work is a derivative of Medicines by Design by US Department of Health and Human Services, National Institute of Health, National Institute of General Medical Sciences and is available in the public domain. ↵
- Fernandez, E., Perez, R., Hernandez, A., Tejada, P., Arteta, M., & Ramos, J. T. (2011). Factors and mechanisms for pharmacokinetic differences between pediatric population and adults. Pharmaceutics, 3(1), 53–72. https://doi.org/10.3390/pharmaceutics3010053 ↵
- Fernandez, E., Perez, R., Hernandez, A., Tejada, P., Arteta, M., & Ramos, J. T. (2011). Factors and mechanisms for pharmacokinetic differences between pediatric population and adults. Pharmaceutics, 3(1), 53–72. https://doi.org/10.3390/pharmaceutics3010053 ↵
- "Grapefruit" by ExplorerBob is licensed under CC0 ↵
- This work is a derivative of Medicines by Design by US Department of Health and Human Services, National Institute of Health, National Institute of General Medical Sciences and is available in the public domain. ↵
The administration of antimicrobial drug therapy involves special considerations to ensure that the therapeutic drug effect is achieved while maintaining patient safety and minimizing complications.
Let's consider some of the variables that may impact antimicrobial administration:
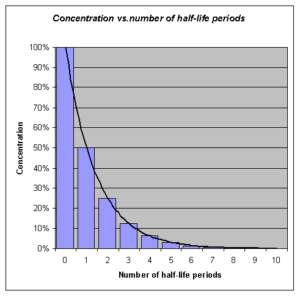
Half-Life
Many antimicrobial medications are administered to ensure a certain therapeutic level of medication remains in the bloodstream and may require interval or repeated dosing throughout the day. For example, the , or rate at which 50% of a drug is eliminated from the plasma, can vary significantly between drugs. Some drugs have a short half-life of only 1 hour and must be given multiple times a day, but other drugs have half-lives exceeding 12 hours and can be given as a single dose every 24 hours. Although a longer half-life can be considered an advantage for an antibacterial when it comes to convenient dosing intervals, the longer half-life can also be a concern for a drug with serious side effects. Medications that have longer half-life and more concerning side effects will exert these side effects over a longer period of time.
See Figure 3.6[1] for an illustration of half-lives and the time it takes for a medication to be eliminated from the bloodstream.
Lifespan Considerations
A majority of medications are calculated specifically based on the patient's size, weight, and renal function. Patient age and size are especially vital in pediatric patients. A child's stage of development and the size of their internal organs will greatly impact how the body absorbs, digests, metabolizes, and eliminates medications.
Liver & Renal Function
Additionally, there are many antimicrobial medications that will require tailored dosing based on individual patient response and the potential impact of the medication on the patient's liver and renal function. For more information about the effects of liver and renal function on medications, refer to Chapter 1 regarding metabolism and excretion. Often times, pharmacists and providers will collect drug peak and trough drug blood levels to determine how an individual patient's body is responding to an antimicrobial. Follow-up interval dosing is then prescribed based on these blood levels. This is especially important for older adults or those with known liver/renal impairment. Individuals with diminished liver and renal function are more prone to drug toxicity because of the reduced ability of the body to metabolize or clear medications from the body. For more information about peak and trough levels, refer to Chapter 1 regarding medication safety. [2]
Dose Dependency/Time Dependency
The goal of antimicrobial therapy is to select an optimal dosage that will result in clinical cure, while reducing the patient complications or significant side effects. Many medications may be . This means that there is a more significant killing of the bacterial with increasing levels of the antibiotic. For example, fluroquinolones are dose-dependent medications with the treatment goal to optimize the amount of the drug. Other medications are . Time-dependent medications have optimal bacterial killing effect at lower doses over a longer period of time. Time-dependent antimicrobials exert the greatest effect by binding to the microorganism for an extensive length of time. Penicillin is an example of a time-dependent medication where the goal is to optimize the duration of exposure.[3]
Route
It is also important to consider the route of drug administration within the patient's body. Many of us may have been prescribed oral antibiotics and have simply filled our prescription and completed the drug regimen within the comfort of our own homes. However, there are many types of infections or disease processes that do not respond well to the use of oral antimicrobial therapy. For these diseases, patients may require intravenous or intramuscular injections. Patients requiring intravenous or intramuscular injections may need to be hospitalized, have home health nursing arranged, or travel to the hospital/clinic for their therapy. Concerns with treatment compliance exists with all routes of administration. For more information about considerations regarding routes of administration, refer to Chapter 1 on absorption. See Figure 3.7[4] for an illustration of three common routes of medication within the body.
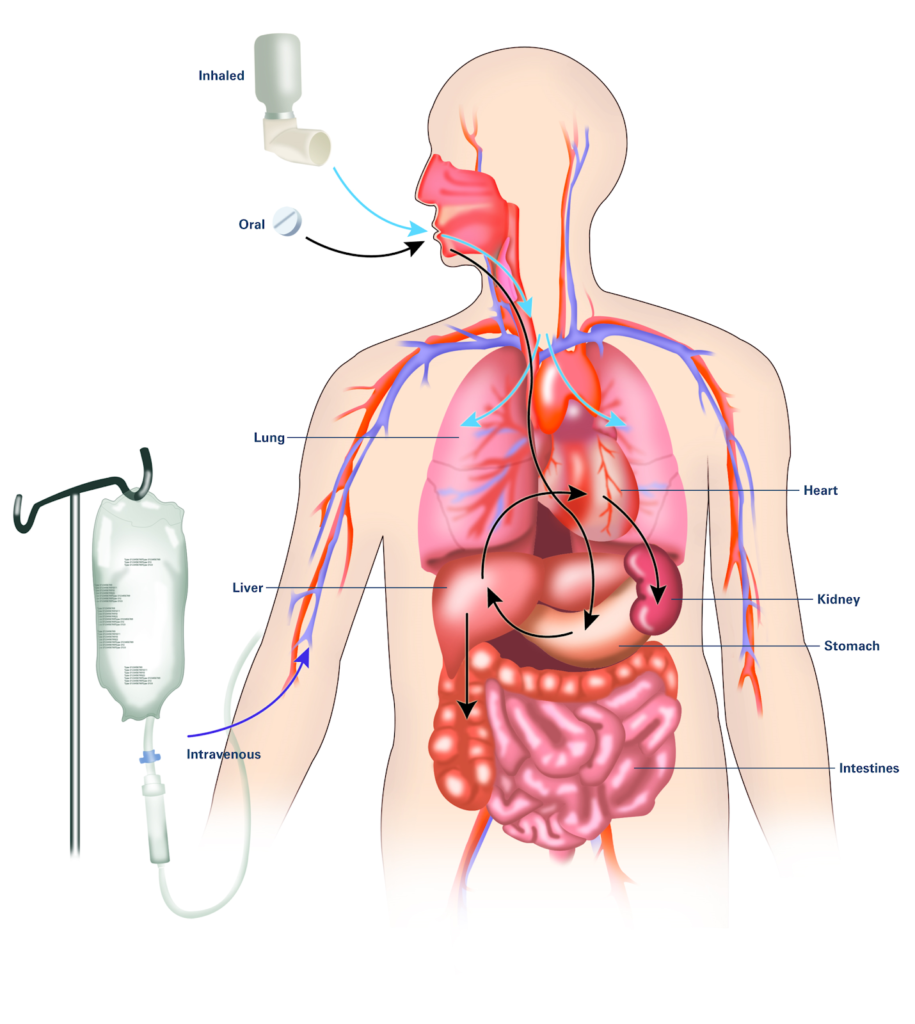
Drug Interactions
For the optimum treatment of select infections, two antibacterial drugs may be administered together. Concurrent drug administration produces a that is better than the efficacy of either drug alone. In this case, TWO is truly better than ONE! A classic example of synergistic drug combinations is trimethoprim and sulfamethoxazole (Bactrim). Individually, these two drugs provide only bacteriostatic inhibition of bacterial growth, but combined, the drugs are bactericidal. [5]
Although synergistic drug interactions provide a benefit to the patient, produce harmful effects. Antagonism can occur between two antimicrobials or between antimicrobials and non-antimicrobials being used to treat other conditions. The effects vary depending on the drugs involved, but antagonistic interactions cause diminished drug activity, decreased therapeutic levels due to elevated metabolism and elimination, or increased potential for toxicity due to decreased metabolism and elimination.
Let's consider an example of these antagonistic interactions.
Many antibacterials are absorbed most effectively from the acidic environment within the stomach. However, if a patient takes antacids, the antacids increase the pH of the stomach and negatively impact the absorption of the antibacterial, thus decreasing their effectiveness in treating an infection.